Scope, inspiration and application of noise reduction technologies
This article aims to address the predominant concerns of noise pollution in urban environments over the past years, as well as to explore strategies for the reduction of noise and to examine future technological developments as potential countermeasures.
Urban noise exists in our lives since many years but has never been so intense and pervasive as in the last decades. The high concentration of citizens in cities of developing countries increased dramatically the frequent use of transportation (including road, rail and air-traffic), construction and public work, ventilation systems, office machines and home appliances. The increasing demand for flights as shown in Figure 1 (with an approximate growth of about 5% per year) approximately doubles the volume of air traffic every 15 years by leading to an increase in the noise levels at the areas at the vicinity of airports. Furthermore, the increasing demand for energy consumption and the global objective of seeking sustainable energy sources have led to a rise in the installation of wind turbines and the size of their blades (see Figure 2). These larger blades generate powerful aerodynamic noise that can propagate over long distances.
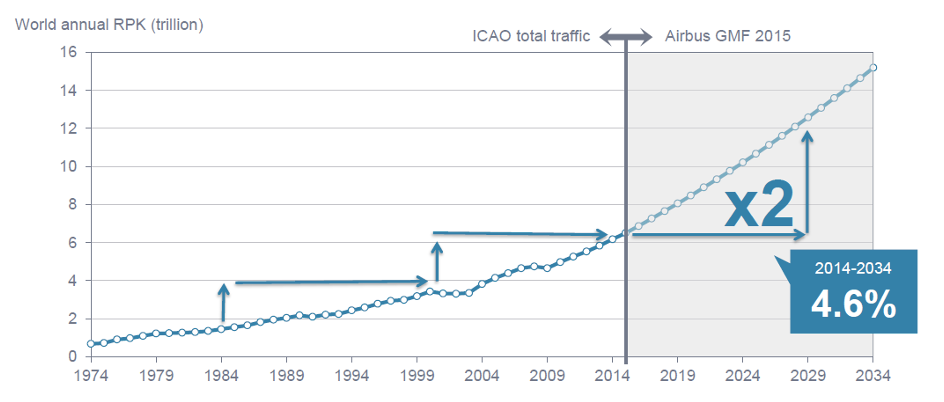
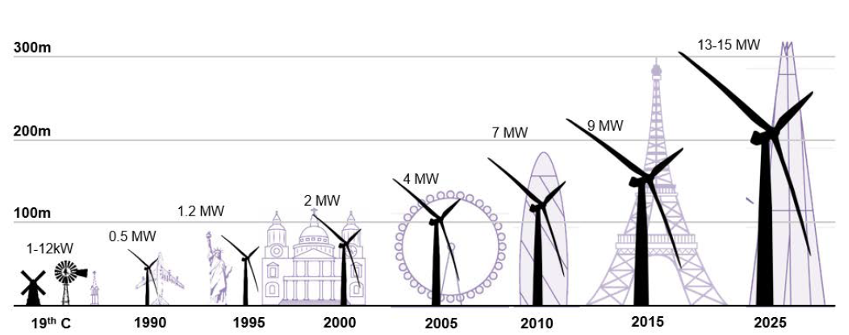
European targets for sustainable development
According to the World Health Organization in 1995, approximately 40% of the European population is exposed to road traffic noise with an equivalent sound pressure level exceeding 55 dB(A) during the daytime, and 20% are exposed to levels exceeding 65 dB(A). Latest recommendations (strong or conditional) have been published for road traffic noise, aircraft noise, wind turbine noise, and railway noise. ACARE has set targets with high expectations within Flightpath 2050 to improve air travel by 2050. Specifically, the perceived noise emission from aircraft should be reduced by 65% by 2050 relative to 2000. It is crucial for society to promptly implement suitable measures in response to these objectives.
What is the origin and nature of industrial noise?
The aforementioned engineering domains partially derive the origin of their noise source from flow-generated sound. Specifically, low-speed fans in engine cooling units, ventilation systems, wind turbines, as well as propulsive rotating-blade technologies for aeronautical transport, drones, and others include these sound sources. In particular, the noise emissions generated by aeronautical and wind turbine technologies reach such significant levels that their mitigation needs to be prioritized and regarded as an urgent issue within the industrial sector.
Over the past decade, industry and academia have collaboratively worked towards a noise mitigation path by studying and developing promising technologies for reducing aerodynamic noise either at its source or along its transmission path. As shown in Figure 3, the fan and the turbine represent the sources, and the blue arrows correspond to the sound transmission path towards the open air. In industrial applications, the ideal intervention would involve noise mitigation both at the source and along the transmission path. However, this is not always achievable due to spatial, weight, and performance restrictions. The inspiration and description of different mitigation techniques and devices are outlined in the following paragraphs.
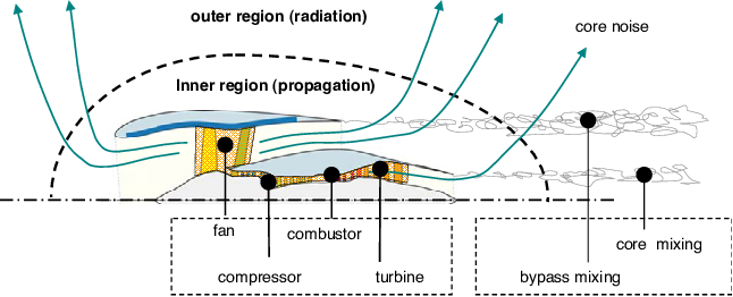
Exploring the inspiration behind noise reduction techniques
Noise mitigation at the source of aerodynamic sound can be achieved through geometrical and structural modifications of the wing shape. These modifications are inspired by nature and have been extensively studied in engineering sciences for their aerodynamic and acoustic effects. The secrets for the noise reduction technology stem from owl wings. Owls possess a distinctive set of wing and feather characteristics that allow them to minimize the sound generated during flight. This effect enables them to fly just inches from their prey without being detected. A particular example among different species of owls is the barn owl, as shown in Figure 4.
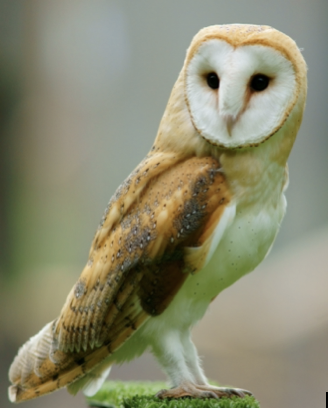
Three special characteristics of owl’s wings have been recognized as noise mitigation devices: the comb-like geometry in the leading edge, the fluffy surface on the bottom side of the wings, and the fringes on the trailing edge of the wing. The leading edge of owl’s wings features spike-like serrations of very small size, which control the growth of the boundary layer over the wings by generating streamwise micro-vortices. This, combined with the fluffy structure of the wing surface, indirectly reduces trailing-edge noise. Serrations are also present at the trailing edge, directly contributing to the reduction of trailing-edge noise. The differences between these structures are evident: the former appears comb-like at the leading edge and fluffy at the trailing edge, while the latter appears straight-edged and rigid.
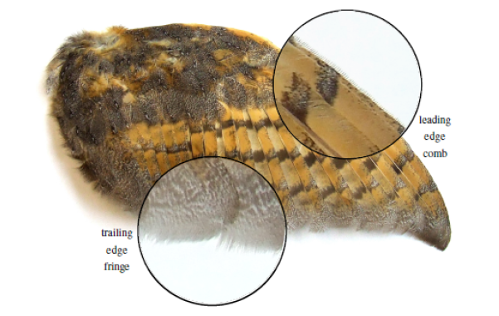
Another source of inspiration is the humpback whale. In contrast to the owl’s comb-like serrations, the foreflippers of humpback whales have leading-edge tubercles with a significantly larger characteristic length compared to the chord, forming a sinuous pattern. These tubercles are known for their high efficiency in hydrodynamics. Unlike other species, humpback whales utilize their foreflippers to propel themselves forward, similar to how birds use their wings for flight, rather than relying solely on their tail, especially during acceleration bursts. One aerodynamic effect of the tubercles is the delay of stall at transient high angles of attack, thereby enhancing maneuverability.
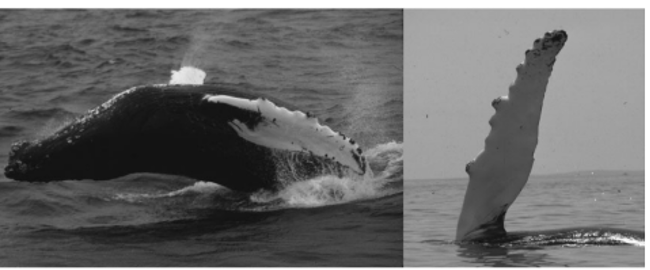
Application on industrial devices
In engineering applications, these technologies are implemented as spanwise-periodic geometrical modifications at the trailing and leading edges, as well as changes to porous surfaces. They have already been applied at turbofan nozzle jet exits and wind turbine trailing edges, as illustrated in the pictures shown in Figure 7. In both applications, serrations are carefully designed to reduce jet noise or trailing-edge noise by altering and mixing the flow patterns downstream of the serrated geometries. The overall noise level reduction achieved in both cases is approximately 2.5-3 dB(A).
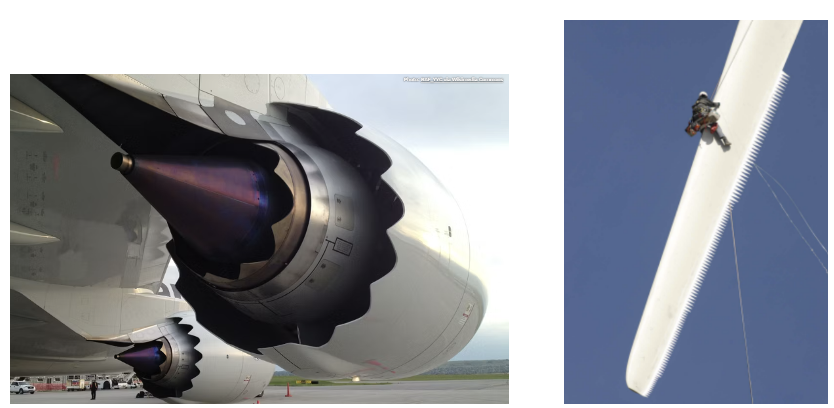
Other industrial applications where serrations can achieve satisfactory noise reduction levels are in drone propellers, outlet guide vanes (OGVs) of turbofan engines, automotive cooling fans, and computer cooling fans. Most of these applications are still in the experimental phase, but they are expected to be available on the market in the near future.
Acoustic liners are another noise reduction technology primarily applied to the transmission path of acoustic waves. In the context of turbofan engines, they are installed within the nacelle walls and consist of multiple layers of sound-absorbing materials, such as porous metal sheets, perforated panels, or composite materials. A typical example of such a liner is a honeycomb structure covered with a perforated panel, which helps absorb sound waves generated by the engine, as shown in Figure 8.
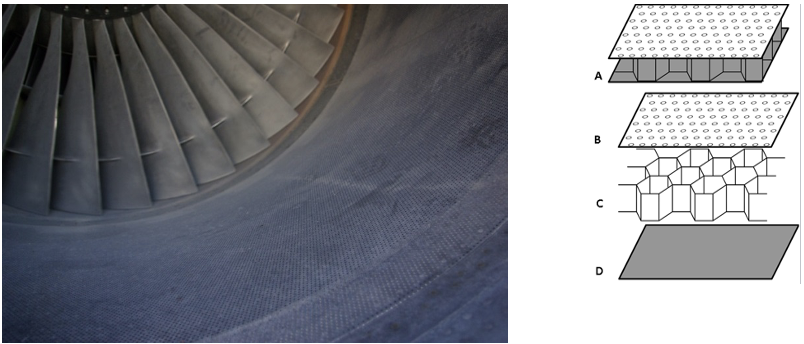
Liners can be also filled with natural or artificial materials depending the application and the engineering environment. Artificially engineered materials namely metamaterials, are designed or ‘tuned’ properly to achieve maximum noise reductions at the frequency range of interest. Samples of these materials are shown in Figure 9.
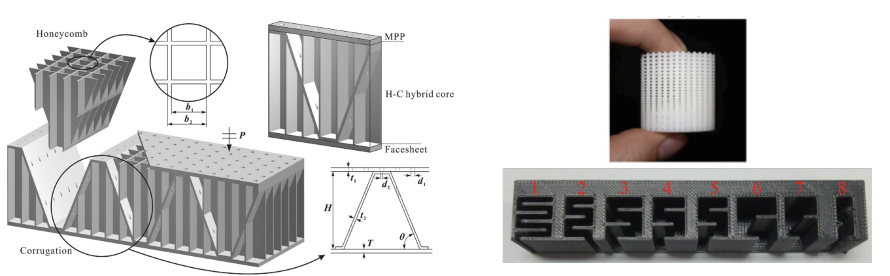
Active and passive noise control
All the above acoustic treatments belong to the passive noise control (PNC) category, in which the materials are custom-designed for fixed noise attenuation performance regardless of the noise environment. There is another noise reduction technique, namely active noise control (ANC) technology, also known as active noise cancellation, which reduces unwanted sound by generating an acoustic signal that destructively interferes with the incoming noise. It involves the use of electronic systems like microphones to capture the incoming noise, signal processing algorithms to analyze and generate appropriate anti-noise signals, and speakers or transducers to emit the anti-noise. It is based on the principle of the superposition of sound waves, where the generated anti-noise wave cancels out the unwanted noise, resulting in reduced overall sound levels. ANC technologies are currently being applied in the automotive sector, primarily with the aim of real-time reduction of aerodynamic and tire noise generated during vehicle cruising. The majority of OEMs in the sound system industry are investing in ANC technology due to its high efficiency and adaptability in various environments with disruptive sound conditions.
In summary
To sum up, this article focuses on the issues of noise pollution in urban areas and explores various strategies for reducing noise levels. It discusses the origins of industrial noise and the inspiration drawn from nature to develop effective noise reduction techniques. Serrated geometries for fan and nozzle applications, acoustic liners, metamaterials, and active noise control technologies have shown to be the most promising noise reduction devices. Through the implementation of these techniques, significant progress can be made in reducing noise levels and creating more comfortable environments for individuals in various settings, ranging from industrial facilities to transportation systems and beyond. In conclusion, by prioritizing noise reduction as an integral part of sustainable development, we can strive towards creating healthier, more livable, and harmonious environments for present and future generations.